Catalytic Carbon Dioxide Hydrogenation to Methane
Carbon dioxide (CO2)is an abundant gas in our atmosphere which could cause further harm to the environment if emissions are left unchecked. Reducing the amount of CO2 released from sources such as gas flues would be highly advantageous in mitigating the negative effects of increased CO2 levels. Unfortunately, renewable energy sources such as solar or wind cannot be scaled like coal to meet varying energy demands. When more energy is needed, more coal is unavoidably burned. However, excess renewable energy could be used to convert carbon dioxide into other chemicals, creating a safe and efficient mechanism for energy storage. This results in less carbon escaping into the atmosphere while producing a useful product. Methane (CH4) can synergize with existing natural energy networks, and in turn be stored and burned when needed. Therefore, researchers are looking for methods to improve the process of converting CO2 to CH4 to make it more efficient.
One of the cost-effective ways of reducing CO2 is through conversion at its sources, rather than capturing it from the atmosphere. The hydrogenation reaction of carbon dioxide to methane, where carbon dioxide and hydrogen react to form methane and water, is promising. Meanwhile this reaction requires significant amounts of energy, typically in the form of heat or light to occur. Catalysts, however, can increase their efficiency, thus raising the conversion of CO2.
Many metal catalysts have been investigated for their effectiveness at improving the reaction to varying degrees of success. Nickel is a cheap and effective catalyst and has comparable conversion to other best performing catalysts. Catalysts also perform far better when paired with a base, which helps disperse the catalyst’s active sites and stabilize them, allowing them to perform better particularly over extended periods of time.
This past summer, I conducted research through the Research Experience and Apprenticeship Program (REAP) to synthesize and test catalysts with varying concentrations of phosphotungstic acid (H3PW12O40, HPA in short). Heteropoly acid has many oxygen atoms capable of dispersing the nickel catalyst, which creates more actives sites, resulting in more CO2 being converted. We also chose titanium dioxide (TiO2), an inorganic powder with a high surface area as a base. It assists by supporting the nickel atoms, which improves the stability and performance of catalysts. The combination of nickel and titanium dioxide has already been studied, but we hypothesized that including heteropoly acids could further increase active sites and improve the conversion of carbon dioxide to methane.
Catalyst Synthesis
During summer 2023, I synthesized several catalysts with varying heteropoly acid percentages. Specifically, I used the sol-gel method to synthesize the HPA-TiO2 support, where titanium (IV) isopropoxide and phosphotungstic acid hydrate are added into a 2-propanol solution. The benefit of this method is to mix the components well, thus generating more active sites throughout the catalyst. I then added nickel before finally calcining HPA-TiO2 support. Calcination is necessary because heating can remove impurities. It was a week-long synthesis process, as many of the steps, such as calcination and drying, take time. I prepared catalysts with weight percentages of 3%, 10%, and 15% of heteropoly acids.
Those catalysts were tested for CO2 conversion. In a typical operation, I mixed the catalyst with quartz sand, then placed it on top of a quartz wool bed in a quartz tube. The quartz sand and wool helped prevent the catalyst from being moved while reactant streams are flowing through catalysts. I attached the tube to a home-made reactor, which consists of a temperature programmed furnace and gas manifold system. Catalysts are pretreated with the diluted hydrogen stream before activity tests. I ran the activity test for varying time intervals under different reaction temperatures.
I used a gas chromatograph (GC) to collect original data needed to calculate CO2 conversion. The GC recorded the amounts of CO2, methane, and other products present at different temperatures. I determined the total concentration of gases by integrating the curves. I ran a baseline with no catalyst and no heat, so the percent change in the presence of the catalyst was able to be measured at different temperatures and compared among different catalysts.
Preliminary Findings
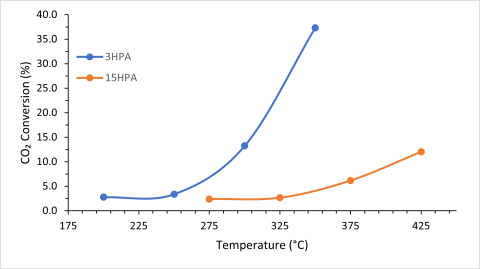
Figure 1: CO2 Conversion over Ni-3HPA-TiO2 and Ni-15HPA-TiO2 catalysts under different reaction temperatures.
My preliminary results showed that the lower percentage of HPA present resulted in higher CO2 conversion rates even at lower temperatures (Figure 1). The catalyst with the highest percentage heteropoly acid resulted in low percent conversion even at a higher temperature, which means initial results point to more HPA having a negative impact on conversion and performance. The catalyst with 10HPA loading was not included due to issues with a gas cylinder low on carbon dioxide, where a negative conversion was found.
I had challenges successfully synthesizing catalysts that had to be resolved. The water concentration from the catalyst with 15HPA loading resulted in no gel forming, which negated the potential benefits to sol-gel synthesis. To ensure my results, I plan to test the catalysts again with different reduction temperatures, reaction temperatures, and for different periods of time, optimizing the testing process for future and current catalysts containing heteropoly acids. The selectivity also needs to be determined, which is the amount of desired product (methane) formed relative to other products of the conversion process. The selectivity results will be used to confirm if other products such as carbon monoxide are present, which are considered undesirable in this study.
Significance of this Research
Carbon dioxide is a large contributor to the changing climate. While CO2 conversion is currently hindered by high energy demands, catalysis possesses the ability to reduce the energy cost, thus making the process more economically viable.
My research is significant due to the scope of the challenge it hopes to overcome. In catalytic CO2 methanation, there are thousands of variables and combinations, each of which warrants testing. Every paper published often leads to even more questions. While the results of my own initial research may not be significant enough for application, it provides insight into a wide scope of potential improvements.
There is still so much more to be assessed. Even a miniscule improvement to current catalyst options could cause significant innovation when built upon by other researchers. I look forward to continuing my research in Dr. Yi’s lab. I would consider different ratios of nickel, heteropoly acids, and base. While the combination tested may not have yielded significant results, there are dozens of others which require testing before any conclusions can be made about the role of heteropoly acids.
I would first like to thank Dr. Nan Yi for his guidance and enthusiastic support as I got introduced to research through my REAP project. Without his knowledge and leadership, my research endeavors would not have been possible. I am also thankful for the other lab members who provided advice and assistance when needed. I would also like to thank my generous donor, Mr. Dana Hamel, through whom my REAP experience was possible in the first place. Finally, thank you to the Hamel Center for Undergraduate Research for awarding me the REAP award; with this research experience, I have gained valuable skills and experience that will continue to propel me towards my academic and industry goals.
Author and Mentor Bios
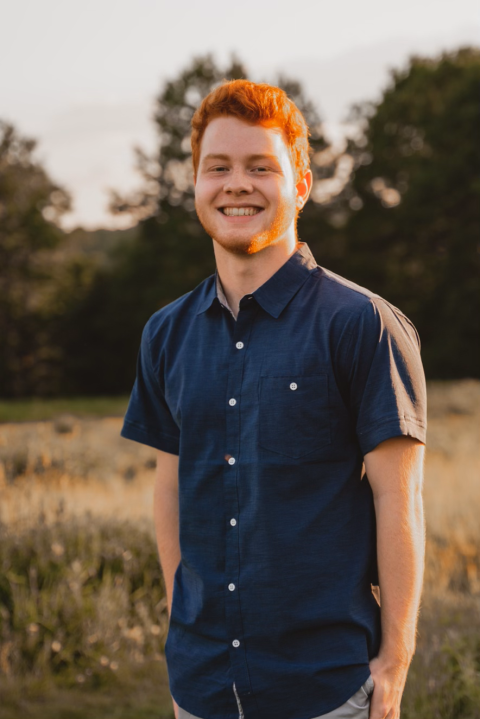
Originally from Barrington, New Hampshire, Sam Lavoie will graduate from the University of New Hampshire in spring 2026 with a bachelor of science degree in chemical engineering and a business administration minor. He is in the University Honors Program, a member of AXΣ, the chemistry fraternity, and a student ambassador for the Hamel Center for Undergraduate Research. Sam has always been interested in human impact on the environment, as well as finding novel ways to cleanly create energy to form long-term solutions. He found a great fit for the Research Experience and Apprenticeship Program (REAP) with Dr. Yi’s lab. Overcoming barriers during the research process made Sam feel confident as he made progress toward an original research question. He believes a major part of research is sharing results with others to be checked and improved upon, which is why he is publishing in Inquiry, completing the full research process. In the future, Sam plans to pursue a graduate degree and is drawn to working in renewable energy or emission reduction.
Nan Yi is an associate professor from the department of chemical engineering and bioengineering at the University of New Hampshire. He leads a catalysis research group which strives to achieve the goals of replacing petroleum derived chemical and fuel production with more sustainable routes. Additionally, his students’ laude his approach to teaching, which integrates research into his classrooms and STEM outreach activity.
Copyright © Samuel Lavoie